Nearly a decade and a half ago, when first starting my Biochemistry Major, I was happily surprised to see that the then newly found knowledge and scientific techniques almost necessarily seemed to spell the end of at least many types of cancer. A foregone conclusion, I remembered thinking….
Quite a few years later, I am very surprised about the lack of progress made along the fascinating track I had envisioned. Needless to say, people continue to die from the ignoble disease. Therefore, it is time to put this concept to paper, enlighten a few people, and perhaps get feedback from experts on what technicalities are holding up the shooting of Paris’ arrow into cancer’s Achilles’ heel. The casual (non scientist) reader will likely find the true nature of cancer and its most logical solution surprising and fascinating; as its true nature is so different from what is most often portrayed.
In first order of course, is an understanding of What Is cancer… The disease’s most broad definition sets it apart from almost all other human diseases. Generally speaking, most human diseases can be categorized in two broad groups; those that are pathogenic, and those that are not. A pathogenic disease is pretty straightforward, and as is well known today, caused by microorganisms (bacteria, viruses, protozoans, etc). These are often contagious and include well known illnesses such as influenza, small pox, malaria and sexually transmitted diseases such as HIV.
Diseases caused by these external organisms typically leave us with a more straightforward (though not always simple) line of attack in the quest for cures; get rid of the pathogens. Your average schoolboy today knows that illness caused by bacteria can often be treated with antibiotics, while those caused by viruses (that many times reflect very similar symptoms) cannot. When little critters are the cause of the sickness, we can either kill the critters or make ourselves immune to them to begin with (vaccination).
Diseases that are not pathogenic, do not lend themselves to such simple treatment, especially when they are genetic in nature (as opposed to bio-mechanical or physiological diseases). This is due to the painful fact that the sickness is not being caused by a foreign invader that must be simply killed, but by a problem or defect within our own bodies. So to illustrate the point in the extreme, while scurvy (caused by lack of vitamin C – often incurred by Age of Sail mariners during long voyages on diets devoid of fruits and greens) can be simply cured by consuming vitamin C, and joint replacement (like hip replacement surgery) may alleviate cases of osteoarthritis much like laser eye surgery can correct nearsightedness; it is quite another matter to attempt to treat something like Down Syndrome or Cystic Fibrosis.
In the case of Down Syndrome for example, the afflicted individual has an extra copy of chromosome 21. Humans have 23 chromosome pairs, one chromosome in every pair inherited from each of the parents. Down Syndrome, thus also known as trisomy 21, occurs when an individual has 3 number 21 chromosomes as opposed to 2.
Human embryos, develop from one original cell – the fertilized egg; the egg and sperm respectively each carrying the 23 chromosomes that combine to form a full set of 23 chromosome pairs (46 total chromosomes). This original single cell, divides, replicating this exact DNA structure inherited from the parents with each division. In essence each cell in the human body (except mature red blood cells), contains the individual’s entire genetic code.

Above are the 22 autosomal chromosome pairs (one inherited from each parent) and the one sex chromosome, well known as XX or XY, determining the individual’s sex.
Thus, in the case of genetic disorders, every cell in an individual’s body contains the genetic code responsible for the problem. Even if it was possible to alter or remove the problematic gene or genes from one cell, it is an entirely different story to attempt to change the DNA makeup of every cell in an adult human body (very roughly about 50 trillion cells).
In this neat distinction between genetic disorders, and microbial infections, cancer lies on a plane of its own.
Of course, I must note that the reality is quite a bit more complex since pathogens, genetics, the environment and other factors often affect each other (in ways both understood and not yet known) – viruses for example, change their victim cell’s genetic makeup and there is evidence that at least some cancers can be caused by viral infections. Likewise, it is well known that people’s propensity to develop many forms of cancer is itself influenced by genetics, or in other words, hereditary. Needless to say, we are all aware of certain environmental factors (like smoking) that can cause (observed by increased likelihood to develop) cancer. But putting aside the complex relationships between environment, genetics and microbial fauna when it comes to cancer, which is rather well known by the public, we are left with the true essence of the disease which ironically is not well understood by the general public at all.
All the above refers to factors that can cause or increase the likelihood of an individual developing cancer. But what actually is the cancer itself? Well, put simply, a cancerous cell by definition is one whose DNA has been altered and has now gone… berserk. Rather than doing its part as a tiny cog in the wondrous human body, it often divides and spreads indiscriminately and/or engages in other abhorrent behavior which ultimately can lead to the human’s death. Our trillions of cells know when to divide, when to stop, and what functions to perform and not perform in order to maintain the whole system (the person) alive. A cancer cell does not.
Unlike the case with humans, the reason that the cell has “gone postal” is clearly understood… a genetic mutation. The DNA in a cancerous cell is literally different than the DNA inside all the other healthy cell’s inside the organism’s body. As was noted above, in a healthy body, virtually all cells contain an identical set of chromosome pairs (chromosomes are simply long chains of DNA). So unlike the case in a genetic disorder, where all the cells contain the “faulty” DNA, only cancerous cells in an otherwise healthy body contain faulty DNA in the case of a cancer patient.
This difference is crucial; we can also note that similarly, unlike the situation with pathogenic disease, there are no “foreign bodies” to simply try to nuke into oblivion. Cancer is an odd case in between – in a fully formed (and often quite mature) adult, some cells within the body decide to turn into suicidal cells where the genetic code for this change was never present in the body to begin with (at birth).
Why does this happen?
A question that has been and continues to be researched without limit… and the answers are thus far incomplete and complex; but we can set those aside for the moment. The point is that a genetic mutation occurs within a cell that turns into a cancerous cell and it subsequently divides, spreads and grows to often lethal proportions. Once the mutation exists in the first cell, regular cell division continues to copy and replicate the defective DNA into more and more cells.
When certain things are considered to be carcinogenic, what is meant is precisely this: that be it smoking, Ultra-violet rays, X-rays, certain chemicals in food or anything else, exposure to it can cause mutations in the genetic make up of cells.
Presumably, and statistically, such mutations and errors in DNA replication during division occur “all the time” and at random. But statistics comes into play once again. It is likely that most or many small mutations would not cause any real difference in a cell, or at least not in a body of 50 trillion cells. Certainly, as long as it doesn’t divide and spread, it hardly matters what the genetic makeup of say… one lonely skin cell is, it will be pretty harmless to a large organism such as a human. On the other hand, when a mutation is important, and causes a significant change in a crucial gene, it is very often lethal to the cell. In this way, mutations are self-regulatory because most significant mutations would cause the cell’s death. And so, it is a statistically minute sample, that can at the same time receive precisely the mutation that firstly does not kill the cell, and secondly makes it spread uncontrollably in a malignant manner (besides many other requirements of course like the ability for angiogenesis, creating its own blood supply so that the cancer can continue to grow etc).
But statistic games aside, when dealing with trillions of cells, many trillions of divisions and decades of life, regardless of the small chance, presumably all things eventually happen. What is still unknown then is if “cancerous” mutations happen rather frequently in a healthy body, and the healthy immune system simply knows to detect and kill such a cell (which is now as if a “foreign body”), and only in an aging or otherwise unhealthy body the immune system fails to do this, or if rather it is purely a statistical issue where it tends to happen that older people are more likely to develop cancer than young people because their bodies simply had more time to experience this freak mutation.
Some scientists have chosen to focus on this potential difference, because if the former is true, the essence of cancer is not really the cancerous cell, but rather the weakened immune system which normally knows how to kill them to begin with. If this is the case, a potential cure would be to fix whatever is wrong with the immune system.
An example of this thinking, though somewhat misguided, is based on the idea that sharks do not get cancer. Shark cells divide and are exposed to the elements like all other multi-cellular organisms. So if they do not develop cancer, they must have some ability, likely in their immune system, which prevents it from spreading. This has led to a giant industry that sells non effective shark based cancer treatments to desperate patients, and claims millions of shark lives (the latter less of a concern at the Lighthouse). Apparently, it is a myth that sharks do not get cancer, and though they MAY (even this is not proven) have a very low incidence of it, they are also made up of cartilage which may in itself explain it.
Regardless of the cause of the mutations, and regardless of whether a strong immune system ought to and can destroy cancerous cells before they become a threat, a cancer cell is in fact simply a cell that has different DNA than all of its neighboring healthy cells.
The Cure
This fact leads us to the most logical line of attack in respect to a cure. Traditional medical treatments against the disease have been like shooting an anti-tank missile at a fly on the wall. Chemotherapy, radiation treatments, and the like often simply kill human cells, cancerous cells among them. The patients are weakened and often killed by the treatment itself, and the treatment themselves are very carcinogenic… so that if the patient survives this cancer, in time he is likely to develop another due to the treatment.
Obviously the name of the game is to target cancerous cells, and leave healthy cells alone. Antibiotics like the world-famous penicillin work this way since they target and kill bacteria while causing virtually no harm to human cells. Lots of things kill bacteria and other microbes, bleach, fire, strong acid and ether among them, but that would hardly be a cure to anything since they all kill human cells as well. You can disinfect your floor with bleach, but not your patient (if you want him alive). Likewise, an intelligent cure for cancer would target only cancerous cells.
Many scientists indeed, following this rational, have attempted to achieve this type of targeting, but in very surprising and complex ways. In order to target one thing over another, you must first know their difference; and the essential difference between cancerous cells and healthy cells in a patient has been almost ignored. Their DNA.
A cure for any cancer on any patient, would target the mutated cancerous DNA in cancer cells and leave healthy normal cells alone. Almost by definition, malignant cancerous cells have a good blood supply (to stay alive, and to get nutrients to continue to grow and divide), and thus would be easily targeted by a drug in the blood stream. A cancerous cell without blood supply may be shielded from the drug, but so would it be from oxygen, nutrients, waste removal, and other essentials that would render it harmless if not dead.
Personal cure
Why has this been so often overlooked? Because it does not lend itself… to a A CURE… but rather to a personal cure. Though some types of cancer are caused by well known standard mutations, others are quite “personal”. Beyond this, the exact difference between a healthy cell’s DNA, and a cancerous cell’s DNA in a cancer patient is almost always “personal” because virtually everyone’s DNA is a bit different to begin with. That is what makes you… you, as opposed to someone else.
This fact at first sight, causes some serious obstacles in the quest for a cure… since a single standard cure may not be possible, even for common cancers. But this has led scientists in quests riddled with even greater obstacles… the quest for a single CURE to a very personal disease. The fact is, that recognizing how personal a given cancer is, with today’s technology, would render a personal cure much more plausible and effective.
There are various ways that such a cure can be approached, as long as the crux of the matter is recognized, that it is a personal cure for a specific patient and his specific cancer. Here is but one example of the mechanism involved.
The first step is to find out precisely what the mutation, in essence the genetic difference between the cancerous cells and the healthy cells, in the patient’s body is. Not too many years ago, this along would be a task of monstrous proportion, especially for only one patient. Let’s recall The Human Genome Project, initiated under President Reagan in 1987, and scheduled for 15 years, it was seen as an extremely ambitious and enormous project. It’s goal was to map the Human Genome. Whose genome? A few anonymous donors were chosen. The project was officially completed in 2004, and today, there are hundreds of full human genomes publicly available that have been fully sequenced.
The cost, resources and time that sequencing a full human genome requires continues to drop with increasing technology. Quite famously, Steve Jobs had his full genome sequenced for only $100,000 USD. With today’s Health Care costs, and relative to other cancer therapies, one hundred thousand dollars is not a particularly large figure. Obviously, if done on a larger scale, the price would continue to drop. Furthermore, full genome sequencing may not be required for at least many types of cancer patients, as methods more similar to DNA matching and DNA fingerprinting may suffice.
We are all aware of how experts involved in criminal investigations or trials, routinely compare human DNA found in crime scenes to that of potential suspects. Similar methods are used in population genetic studies, genetic archaeology, paternity tests and other situations. In essence, the goal in all of these cases is to find the differences if any, between two sets of human DNA. That is precisely the same as what is needed in our cancer cure.
So be it with DNA fingerprinting which can more efficiently zero in on genetic differences, or at worse case, full genomic sequencing, today it is already perfectly possible to find out the precise difference between a patient’s normal genetic makeup, and that contained in their malignant cancerous cells.
Some Technicalities
For the uninitiated, let us clear up what we are referring to. What is sequencing, and what is DNA? How is one DNA different than another. Without delving too deeply into technicalities, DNA, is a molecule made up of the nucleotides guanine, adenine, thymine and cytosine. These four nucleotides are linked together in very long chains, and in any order. It is essentially their order that “codes” for genes and other genetic information. Scientists refer to the four nucleotides as G, A, T and C for short. To make matters slightly more complex, the nucleotides or “base pairs” as they are also known, not only link vertically with other nucleotides to form the long chain, but also horizontally with a partner nucleotide to form the base pair. In this way, the two long chains are linked to each other to form the famous DNA double Helix (essentially a chromosome).

The illustration shows the nucleotide base pairs, forming the well known DNA double Helix.
Therefore, if we take any single chain of the double helix, and figure out its nucleotide sequence, “A-T-C-C-C-C-G-T-T-A-T…” for example, we have “sequenced” the DNA. The genetic code is therefore a variation of the four base pairs or nucleotides existing in DNA. Having said that, it is important to keep in mind, that most people’s genetic code, when sequenced in this way, is extremely similar. An average human and an average chimpanzee, will share roughly 95%-98%% of their DNA. And individual humans, will tend to share 99.9% of it.
It is this fact that makes DNA fingerprinting so impressive, and it is the same tools that could be used to find the minute differences between a patient’s cancerous DNA and its healthy DNA. DNA fingerprinting uses techniques such as PCR and DNA splicing to find differences in the genome.
Full genome sequencing on the other hand, is the decoding of the entire DNA content of an individual or organism… in the case of human’s the entire DNA makeup of a single cell would be about 3 billion base pairs long!
Delivery
Today, hundreds or even thousands of individuals have had their full genomes sequenced. Furthermore, in 2008, researches at Washington University in Missouri, published a paper comparing the exact genetic differences between an acute myeloid leukemia patient’s malignant cells and normal healthy ones. The fascinating results indicate 10 exact differences (mutated genes) between the two genomes. Two of them were previously known as mutations contributing to the cancer, and 8 were completely novel.
A targeted therapy does not to target ALL the mutations, but only some or even only one at minimum. The idea is to find a a sequence that is unique to the cancerous cells, and is not present in the healthy cells. For example, let us say that the healthy cells contain a specific sequence in some part of their genetic makeup:
A-C-C-C-C-C-C-T-T-G-A-A-G-G-C
The above sequence is never repeated anywhere else in the genome. This is an important requirement, as the therapy will target all instances of a certain sequence. In the cancerous cell, this unique sequence is mutated to:
A-C-C-C-C-C-G-T-T-G-A-A-G-G-C
This sequence also is unique in the entire genome. We now have a target for our therapy. All cells that contain the above genetic sequence anywhere in their genome should be targeted for destruction. The idea is to use statistical modelling, once the genomes have been sequenced, to find the smallest unique sequence found in the cancerous cells that is NOT found in the normal healthy cells.
How can they be targeted? Well, nature here gives us the answer. DNA by its very nature is meant to be “accessed” or “used” by its specific sequences; that is how it can “code” for information (such as proteins) and have an actual use. Otherwise, its just a long molecule of nucleotides which in itself, does nothing. The human body is already filled with proteins and other molecules that attach and interact with DNA at certain sequences. Basically, molecules can be shaped so that they “fit” only when bumping into a specific DNA sequence; much like a lock and its key.
Transcription factors are proteins that bind to certain DNA sequences (such as enhancers, promoters, silencers, etc) and regulate gene expression. They may activate, promote or repress the expression of a certain gene. For example, a classic example is in the simpler prokaryotes, the lac operon of the bacteria E. coli.
E. coli contains a gene which codes for the proteins (enzymes in this case) lacZ, lacY and lacA (β-galactosidase, lactose permease, and thiogalactoside transacetylase respectively). These aid in the digestion of lactose (milk sugar). From an evolutionary standpoint, it is a waste of energy and resources for the bacteria to produce these enzymes if lactose is not an available food source. Though bacteria can consume milk, most bacteria are not living on milk at any given moment. Therefore, it would be advantageous to the bacteria if it could produce these enzymes when necessary for lactose digestion, and not produce them when lactose was not available.
This is what the lac operon basically does. Before the coding for the first gene, lacZ, there is a short and unique DNA sequence called the lac operator. A regulatory protein called lactose repressor binds to this operator, and in turn inhibits the transcription of the genes. The repressor gets in the way of RNAP binding to the promoter sequence which helps initiate mRNA transcription of the gene.
When lactose is available in the medium, allolactose (a lactose metabolite) binds to the repressor protein and alters its shape. Once altered, it cannot bind to the operator, and in turn does not inhibit the promoter and the genes are expressed freely. In eukaryotes such as humans, these regulatory sequences and proteins, work in complex, varied and not yet fully understood ways, but the principle being illustrated here is straightforward. Proteins exist (and can be designed) that bind to specific DNA sequences. The binding area of the protein is called a DBD. In addition to the DBD, the protein can have other varied and large parts, extending to adjacent space, and therefore blocking the access to other neighboring DNA sequences, in effect just like the lactose repressor blocks access to the promoter in the bacteria Escherichia coli.
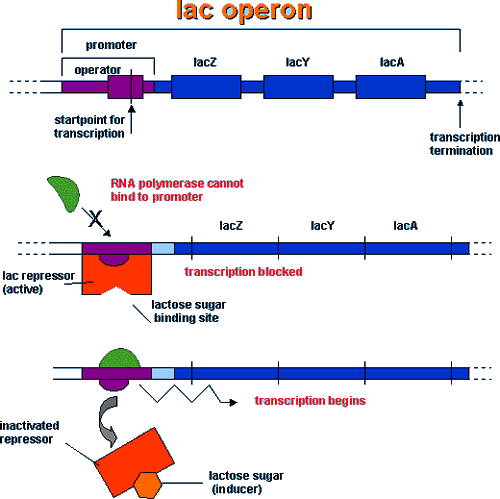
The classic example of the lactose repressor inhibiting RNA polymerase from binding to the promoter (and thus transcribe the gene for protein synthesis). When lactose is present, the sugar deactivates the repressor which cannot bind to the DNA sequence, and allows RNA polymerase to bind to the promoter (and thus the enzymes are produced).
In addition, there are many different molecules in the human body that bind to specific DNA sequences including nucleases (that cleave DNA), histones and polymerases. Restriction enzymes for example, can cleave DNA at specific sequences, and are well known and studied. Over 600 restriction enzymes are currently commercially available for targeting different sequences. Zinc fingers and restriction enzymes (or their nuclease domain) can be combined to target new specific DNA sequences.
The general idea is that if certain proteins can bind to the specific DNA sequence contained in the cancerous genetic code, they can then either interfere with large portions of their genetic code on either (or both) sides, upstream and downstream as it is known in molecular biology, and render the cell either unable to further divide or sustain itself alive, or can simply target these sequences and cut the DNA chain and also killing the host cell. The same proteins would be harmless for the non cancerous cells, which posses no matching DNA sequence.
The custom tailored cure includes two complex and expensive steps, neither of which remains outside of today’s scientific capabilities. First is the decoding of parts (or much of) the patient’s genome and its cancerous counterpart to ascertain the specific culprit mutations.
Secondly, once knowing the genetic differences between the cancerous and healthy cells, designing a specific protein or proteins that will target only the patient’s cancerous cells. At the moment, both of these procedures remain very expensive and time-consuming, but placed in the context of the ongoing costs of current cancer therapies, they are not unreasonable.
Furthermore, as these personal customized cures would be developed in ever increasing numbers, not only would their cost drastically decrease (market pressures, economies of scale, increased efficiencies, improved methodologies etc), but the need to conduct fully “personal” cures would also decrease.
As the database of cancers, and their respective “cures” grows, we will undoubtedly find as we already suspect, that many types of cancers are very similar…. the exact mutations leading to it repeating themselves in many patients. As this database grows, many types of cancers will be easily recognized as one of the “standard” types, and a previously designed “cure” can be applied. This obviously will greatly reduce costs as well.
Possible Technical Obstacles
The main obstacles with this strategy currently lie in the protein (the cure) design. Obviously, some patients’ cancers will lend themselves for easier targeting than others, based on the specific DNA sequence and its length that has to be targeted. An other possible problem will be the protein’s size, and if access to the DNA within the cell’s nucleus will be a problem. The nucleus tends to keep out many large molecules and proteins.
These obstacles are not a deal breaker, and can be overcome with technology and experience. Even if accessing the nucleus in certain instances is impossible, there are other indirect ways of targeting the cancerous cells, once we know its full genetic fingerprint. The malignant DNA may code for very specific mRNA (that goes out of the nucleus to code for proteins) that can be targeted. The protein/mRNA compound can be designed to be lethal to the cell in other ways. Ultimately, the coded proteins can be targeted as well (as opposed to the DNA or mRNA).
The Political Angle
At the Lighthouse, we never lose sight of the political angle of course, and like in so much of life, the government here can indeed be a problem. The above personal cures to the devastating disease that is cancer can be developed only if the forces of innovation and the free market are unleashed. The individuals and companies that must invest great effort, capital and time in developing these must not only be free (legally) to do so, but must have their fruits protected by law, and their profits stolen in the name of social justice.
With a disease like cancer, and the potentially high (at least initially) cost of these specifically designed “personal cures”, companies are understandably afraid that their innovations will be co-opted by governments who will claim everyone is “entitled” to a cure for cancer which did not even exist before its makers created it. These very real fears, keep companies from investing the resources needed for such advances.
Perhaps most devastating is the arbitrary regulatory purgatory that biotechnology and pharmaceutical companies must face with new drugs. The FDA for example, is in no way ready to face this kind of advanced personal custom tailored care. The FDA is set up to delay, and test the launching of new drugs for years if not decades before regulatory approval. One of the cornerstones of this control is the purity and uniformity of the drug; all patients must be receiving an identical drug and exact approved doses. Developing a specific cure for a specific patient’s cancer, would be currently impossible in the current regulatory environment.
This indeed may be one of the major factors why a decade and a half after I saw the end of cancer in sight, so many millions have continued to die at its hands. In the name of social justice and public safety, the government and society along with it is always willing to forget that the things that they believe every person is entitled to, exist in the first place only because of the ingenuity, effort, courage and creativity of those who created them.
It is easy to say that everyone is entitled to health care, but quite another to suggest that doctors and nurses should work for free. Of course they should not work for free you say? Then who is it that should work for free, so that we can take from him and pay the doctor who obviously should get paid for his hard work and many years of study? It is likewise easy to curse the pharmaceutical company for its large profits, and forget that they created the very cures many now believe they are entitled to… simply by the fact that they both (the person and the cure) exist.
When all genius is stolen in the name of social justice, no one will suffer more than the very people in whose name the theft is committed; like with all hidden taxes, we pay them unknowingly… in the case of medicine, with our lives in the absence of cures that were not created by those who were either barred from doing so, or could but would not have them stolen.
Sadly, many go happy to their graves knowing that their would-be saviors were not allowed to have more than they.
Interesting and very clear—well done.
I’ve just come across an article that might shed some light as to why this problem may actually be more difficult than anticipated, even with the wonder of DNA sequencing. http://arstechnica.com/science/2013/06/cancer-gene-sequencing-effort-struggles-through-waves-of-false-ids/
Thanks Yony.
Great find as far as the article. Thanks! Firstly, it is more evidence of how ever simpler, faster and more cost effective sequencing is becoming. Also, it certainly illustrates some of the real-life complexities that I simplified a bit here for the sake of clarity. My paper here is intended for the casual reader. Cancerous cells seem to mutate at a much higher rate than normal cells, and they also apparently tend to mutate and disable genes that themselves inhibit cells that have mutations from replicating their DNA (and thus spreading). These and other similar features are all though, in some way, expected even if not well understood… as I pointed out, a cancer by definition has the very rare and specific mutations that will allow it to become a “successful” cancer rather than just another mutated cell that can either die on its own, not spread, or fall prey to our immune system. Evolutionary (natural selection) pressures work against us when it comes to cells within a larger organism.
The article you bring up however, in another way falls prey to the group-think that I am referring to in my paper. The focus in the study, as is in oncology in general, is to find and understand the relevant cancerous genes and/or mutations. Notice the crux of the article, the study and the project is that many of mutations in cancerous cells are not “relevant”. For example, they narrow down over 400 mutations down to 11 significant ones, of which 10 were already known as cancerous mutations. They propose, that since cancerous cells tend to have many mutations, they carry important ones as well as many insignificant ones.
My angle is that most if not all of those 450 mutations ARE very relevant (as long as they are unique and not present in large number of healthy cells as well). Rather than understanding the inner workings of the cancer, and what specific mutations do or don’t do (which is very important in its own right), I propose to target the very mutations! They want to understand what specific cancer genes do, so that then they can “develop therapies” that target those differences. That is why an insignificant mutation for them is one that doesn’t “do” anything. I propose that a very simple and direct difference is right in front of our noses; the very mutation itself is a physical difference between cancerous cells and healthy ones. If you have a collection of hundreds of mutations to chose from, all the better…. the mutation you target does not have to be important for the cell, it just has to serve as an identifier. Once we target one cell over another, it is easy to kill in a myriad ways. The differences they are looking for are molecular, perhaps a certain protein if they are very lucky, and the difference I point to is also molecular, a DNA molecule. The only thing that makes my target a bit more challenging is that the DNA is in the nucleus, whereas their protein (if it even exists in most, many or even any cases) MAY be in the cytoplasm or cell membrane. This is not an impossible challenge.
You don’t really seem to understand much about biology if you think it should be simple to create something that will only affect those cells with a specific base sequence change without any other difference.
Thanks for the illuminating comment bioturbonik.
The word “simple” is mentioned only twice in my lengthy article. Both times used to describe something that was not simple (at least not in the case of cancer).
and for non-pathogenic diseases:
I did use “simpler” to describe prokaryotes as opposed to eukaryotes, and in one of my above comments but not in the article said that DNA sequencing was becoming simpler (cheaper, faster, etc).
The very beginning of the article however, does invite expert opinion on what of some of the difficulties may be to the explained approach (and the article itself talks about some of the difficulties), so enlightening explanations along those lines or even simple disagreement is most definitely welcome. Baseless personal attacks, less so.
We do always welcome comments from our liberal friends though, thanks.
Good job! Maybe the rich cancer patients need to lead the way towards this treatment. You mentioned that Steve Jobs had his genome sequenced. He probably would have also funded a personal cure if the option was offered to him. If it works at high costs, it should also be able to work at reduced costs as you said.